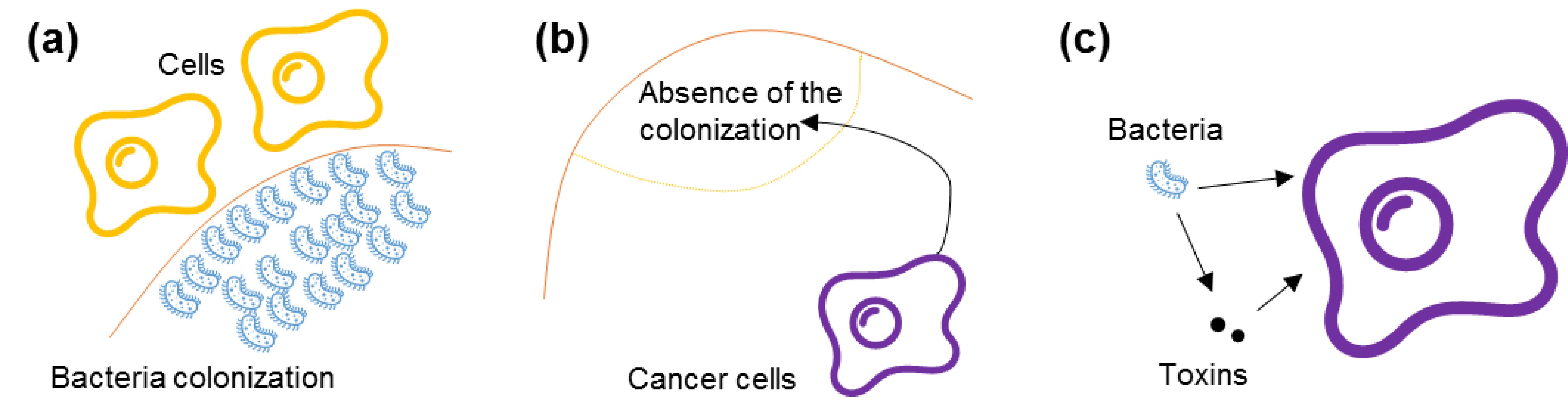
It has been a long-standing interest in the biomedical field to delineate pathogen‒host cell interactions. The latest advancements in single-cell analyses with multiomics approaches have begun to revolutionize our understanding of the impact of intratumoral bacteria on tumor development. Recent studies suggest that intratumoral bacteria modulate the communication between tumor cells and surrounding immune cells, which changes tumor progression and plasticity. Thus, a better understanding of the molecular mechanisms underlying intratumor bacteria-elicited pathogen‒host interactions will shed light on targeted interrogation in clinical oncology. This essay highlights recent progress in intratumor bacterial signaling and host cell plasticity control. In addition, we provide perspectives on how the molecular delineation of intratumor bacterial signaling and host cell plasticity control can help precision medicine and novel therapeutic development.
The emerging networks of intratumoral bacteria-cancer cell communication can be used as targets for precision interrogation of cancer progression.
It has been a long-standing interest in the biomedical field to delineate pathogen‒host cell interactions. The latest advancements in single-cell analyses with multiomics approaches have begun to revolutionize our understanding of the impact of intratumoral bacteria on tumor development. Recent studies suggest that intratumoral bacteria modulate the communication between tumor cells and surrounding immune cells, which changes tumor progression and plasticity. Thus, a better understanding of the molecular mechanisms underlying intratumor bacteria-elicited pathogen‒host interactions will shed light on targeted interrogation in clinical oncology. This essay highlights recent progress in intratumor bacterial signaling and host cell plasticity control. In addition, we provide perspectives on how the molecular delineation of intratumor bacterial signaling and host cell plasticity control can help precision medicine and novel therapeutic development.
Microbial roles in cancer formation, diagnosis, prognosis, and treatment have been debated for centuries. Early studies showed that approximately 16.1% of cancer development in humans is associated with infectious agents[1]. Intratumoral bacteria, as an important part of intratumoral microbes, have been found and identified with 16S rDNA PCR analysis[2]. Infection with Helicobacter pylori (H. pylori) is the most clearly identified risk factor for gastric cancer—the third leading cause of cancer mortality worldwide in men and the fifth in women. The risk of gastric cancer involves interactions among H. pylori strain–specific virulence factors, patient genotype, and environmental factors[3]. For a long time, the study of intratumoral bacteria has remained challenging due to their low biomass. In 2020, Nejman et al.[4] successfully investigated intratumoral bacteria in 1526 tumor samples, including breast, lung, ovarian, pancreatic, melanoma, bone, and brain tumors. Their findings shed light on the role of intratumoral bacteria in tumor development and provide new directions for cancer research. While it has been linked to various cancers, the biological function of intratumoral bacteria remains undefined. Recent findings suggest that these bacteria primarily affect tumor tissues through indirect means, such as metabolites and the immune system, whether located near or distant to the tumor[5]. Recent electron microscopic analysis revealed that only 2%–3% of primary tumor cells contain bacteria, which suggests that tumor cell clusters containing intratumoral bacteria may have a greater survival or selective advantage during invasion, migration and intravasation in the circulation[6]. Intratumoral bacteria regulate various functions of the tumor-bearing meta-organism, primarily through immunomodulation[3–5,7]. Known microbial mechanisms can manipulate nonhematopoietic and hematopoietic components of the gut epithelial barrier, modulate primary and secondary lymphoid organ activities, and regulate the immune tone of the tumor microenvironment (TME). However, the abundance of intratumoral bacteria is low compared to that of cancer cells, and their functional repertoire and potency remain elusive. Given the low number of bacteria-containing primary tumor cells[6], it is possible that only unique cell populations absorb external microbes or obtain a benefit from resident intratumoral bacteria. Thus, this review is focused on the role of intratumoral bacteria in cancer pathogenesis and progression.
For a prolonged period, research on cancer induced by infection has mainly focused on viruses. The exploration of viral pathogens has also been ongoing[8]. Traditionally, bacterial infections have not been regarded as a significant cause of cancer. However, recent studies have demonstrated that two mechanisms underlie the pathogenesis of cancer elicited by bacteria: induction of chronic inflammation and production of carcinogenic bacterial metabolites[9]. The duration of the inflammatory process directly correlates with the likelihood of malignancy[10–12].
Gut bacteria can produce carcinogenic metabolites through several mechanisms. First, bacteria deconjugate and reduce bile acids, leading to the production of cytotoxic 7α-dehydroxylating bile acids (deoxycholate and lithocholate)[13]. These compounds promote cell proliferation[14] and adenoma growth[15], which enhances the carcinogenic effects of exogenous or endogenous mutations. Second, bacteria activate exogenous mutagen precursors, such as fecapentaenes, which are highly concentrated in human feces, but their relationship with cancer has yet to be established[16–18]. Last, bacteria ferment polysaccharides and glycoproteins into volatile fatty acids. These factors may alter the membrane structure, increasing the proliferation of epithelial cells in the distal colon, although direct evidence requires confirmation, possibly in 3D organoids[19,20].
Additionally, intratumoral bacteria can exacerbate difficulties in treatment. Certain bacteria can increase cell resistance to chemotherapy drugs. Colorectal cancer patients with recurrence postchemotherapy contain abundant Fusobacterium (F.) nucleatum, which is related to the clinical and pathological characteristics of the patients. F. nucleatum targets TLR4 and MYD88 innate immune signaling and specific microRNAs, activating the autophagy pathway and hampering the colorectal cancer chemotherapeutic response. Thus, F. nucleatum promotes the mechanism of tumor chemotherapy resistance[21]. Additionally, in colon cancer models, bacteria metabolize gemcitabine (2′,2′-difluorodeoxycytidine), a form of chemotherapeutic drug, into the inactive form 2′,2′-difluorodeoxyuridine[22].
The relationship between bacteria and cancer cells is a complex and longstanding question in the field, given their dynamic and intricate interactions. William Coley’s discovery in the early 20th century of a patient with a sarcoma on his left cheek, who experienced tumor regression after developing a high fever due to a Streptococcus pyogenes infection, strongly suggested that bacteria could inhibit cancer progression[23,24]. However, the mechanism by which bacteria suppress cancer progression remains unclear. In a separate report, individuals predisposed to S. typhi exhibited a higher risk of gallbladder cancer, and some of these individuals lacked IL-12Rβ1 chain expression, which may impair T-cell-mediated immunity[24,25]. Fig. 1 illustrates the steps involved in bacterial interaction with tumors.
Cancer cells can also affect the normal human microbiota. Recent studies have demonstrated dynamic changes in the microbiota composition of the microenvironment between distal and proximal cancers[26]. However, the microbiota of cancer patients may not necessarily arise directly from cancer cells but rather from other lifestyle factors. For instance, a high-fat or red meat diet has been shown to modify indigenous microbiota in CRC[27]. Nevertheless, studies suggest that the unique human microbiota of cancer patients can be used as a marker to detect some cancers by combining several bacteria or microbiota characteristics in a patient’s stool microbiota[28–30].
Multiple factors can affect the intricate relationships between bacteria and cancer cells, including the location of the bacteria. Recent data highlight that the intratumoral bacteria within a tumor are not randomly distributed but rather highly organized in microenvironments and promote cancer progression of development, metastasis, immunosurveillance and chemoresistance[31]. One role of intratumor bacteria is inhibiting Rho-GTPase activity and its influence on the actin cytoskeleton to strengthen cell resistance to high fluid shear stress present in vascular channels[6,32]. These stromal changes, known as desmoplasia, further progress tumor formation with the recruitment of fibroblasts and tumor-related macrophages[33–35]. Thus, the ability of intratumor bacteria to resist environmental mechanical challenges could facilitate the migration of tumor clusters through the stiff tumor ECM. In primary tumors, collagen-rich areas have the second highest population of infected cells (15%), likely CAFs, central cells that control collagen-rich tumor ECM production and remodeling. CAFs exist in blood CTCs and affect the collective migration of tumor cells. If CAFs contain intratumoral bacteria, they could play important roles in regulating metastasis[6]. Tumor-associated macrophages can influence cancer progression and metastasis, but the mechanism remains unclear. Chen et al.[36] found that breast tumor-associated macrophages abundantly produce CCL18, and its expression in blood or cancer stroma is associated with metastasis and reduced patient survival. Interestingly, PITPNM3 was identified as a functional receptor for CCL18 that mediates the effect of CCL18 and activates intracellular calcium signaling. It is of great interest to model the functional regulation of the tumor microenvironment on metastasis using 3D organoids[37].
A previous study showed that inflammation is a key factor in the development of breast cancer in humans[38]. Fig. 2 shows their possible relationships. The microbiota residing on the mucosal surface plays a crucial role in immune system development. However, people chronically infected with pathogenic gastrointestinal bacteria may develop inflammation and cancer. Damage to the intestinal epithelial barrier in such patients causes bacterial displacement to the submucosal layer, continuously activating immune inflammatory response cells, including dendritic cells, macrophages, granulocytes and lymphocytes, ultimately leading to a systemic inflammatory response. This response may occur at extraintestinal sites, such as the breast, resulting in breast cancer. Immunocompetent hosts typically respond to microbial invasion with regulatory T cells and downregulate inflammatory responses to restore intestinal epithelial homeostasis. Conversely, immunocompromised hosts produce excessive inflammatory cytokines, such as TNF-α, which may result in tumor formation. In C57BL/6 Min and Rag2-deficient Min mice, adenosquamous carcinoma occurs in the inflammatory region of the mammary duct and ductal proliferative epithelium, resembling the characteristics of female breast malignancies involving terminal ductal lobular units. Oral and gastric infection with Fasciola hepatica prompts low-grade and high-grade hyperplasia of the breast epithelium, accompanied by the accumulation of mast cells, neutrophils and macrophages around the ducts of infected Rag2-deficient Min mice, indicating their potential involvement in tumorigenesis. Intestinal bacteria can trigger mammary carcinoma, suggesting that an imbalance in the host immune response to intestinal bacteria may influence the development of parenteral cancer, such as mammary carcinoma[39]. Tumor cells can present peptides originating from intracellular bacteria, promoting immune reactivity. Research into intratumoral bacteria of melanoma suggests that bacteria that colonize melanoma tumors can enter melanoma cells, and their peptides can be presented by HLA-I and HLA-II molecules of melanoma cells[40].
A recent study by Cai et al.[5] indicated that bacteria also play an important role in the metastasis of breast cancer cells. Metastasis is a significant and critical stage of tumor development. During the metastasis process, intratumoral bacteria enhance resistance to fluid shear stress by reorganizing the actin cytoskeleton, improving the viability of tumor cells[5]. Further studies showed that only specific intratumoral bacteria play such a role. The impact of bacteria on target cells largely depends on cell type and bacterial strain[41]. Cancer cells infected by bacteria invade their surroundings as single cells and attract myeloid cells to the bacterial regions[31], thereby facilitating cancer metastasis.
In addition to facilitating metastasis, intratumoral bacteria also contribute to genetic mutations, thereby becoming a direct cause of cancer. E. coli isolated from breast cancer cells has been shown to damage the DNA of host cells. By using a reporter of DNA damage, phospho-H2AX[42], Cai and colleagues[5] showed that bacteria can induce DNA double-stranded breaks in HeLa cells. E. coli strains, which belong to the B2 phylotype, contain the pks pathogenicity island, which is associated with the production of the genotoxin colibactin. These pks-positive strains trigger DNA double-stranded breaks and chromosomal instability in colon cancer[43–46].
Interestingly, recent studies show that intestinal microbes translocate to the skin and subsequently to the breast tissue[47,48]. It would be of great interest to delineate how microbial translocation elicits the pathogenesis of distal tissue using organoids linked and evaluated by microfluids with semisynthetic biosensors[49].
Nejman et al.[4] conducted a comprehensive study on intratumoral bacteria, analyzing 1526 tumors, including breast, bone, lung and melanoma. Their results suggest that most of these bacteria primarily exist in both tumor and immune cells as intracellular bacteria, the types of which vary among different tumors. Of the classic tumor types, breast tumors are much more abundant in intratumoral bacteria[4]. Additionally, this study reported the specificity of bacterial prevalence, with certain bacterial species enriched in particular tumor types, as shown in Fig. 3[4]. Given the prevalence of intratumoral bacteria in breast cancer, further investigation of these microbes could lead to significant advancements in this field.
Urbaniak et al.[50] examined the microbiome within mammary tissue collected from Canadian and Irish samples. The results of the study highlighted marked differences between the two samples. The main and enriched bacteria were Enterobacteriaceae (8.3% in CAN and 30.8% in IE), Staphylococcus (6.5% in CAN and 12.7% in IE), Propionibacterium (5.8% in CAN and 10.1% in IE) and Pseudomonas (6.5% in CAN and 5.3% in IE). The primary phylum observed was Proteobacteria. Bacterial diversity exhibited significant variation, ranging from 0.8 to 5.2 in Shannon’s diversity index, with an average value of 3.6. Meanwhile, Proteobacteria, as the main phylum in breast cancer bacteria, is specific compared with bacteria in the vagina, oral cavity, bladder, skin, and gastrointestinal tract. Urbaniak et al.[42] provided additional information about the bacteria found in mammary tissue by conducting extensive research on the relationship between the bacteria and the tissue with breast cancer. The results obtained by ALDEx2 showed that Prevotella, Lactococcus, Streptococcus, Corynebacterium and Micrococcus were abundant in healthy patients, while Bacillus, Staphylococcus, Enterobacteriaceae (unclassified), Comamonadaceae (unclassified), and Bacteroidetes (unclassified) were enriched in cancer patients.
Using fresh frozen samples and unaligned RNA collected from a similar patient source from The Cancer Genome Atlas (TCGA), Thompson et al.[51] found that Proteobacteria, Actinobacteria, and Firmicutes were the most enriched phyla in breast cancer. The most prevalent species were mainly Streptococcus, Lactobacillus, and Acinetobacter, with Gluconacetobacter being the most abundant. Therefore, the most abundant bacteria in breast cancer are Streptococcus, Lactobacillus, Enterococcus, Staphylococcus and Pseudomonas. However, the diversity of bacteria was observed to vary significantly between different samples from different patients, regions, or even different time points[4,42,50,51].
Breast cancer is a widespread, malignant disease that particularly affects females[52]. Research has revealed the significant role of carcinogens in cancer development[53]. Inorganic carcinogens, such as arsenic, chrome, and cadmium, have also been identified as potential carcinogens[54–56]. However, the underlying carcinogenic mechanism remains unclear[54]. Considering that bacteria also contribute to cancer[44], it would be of great interest to explore the relationship between these inorganic carcinogens and bacteria down the road.
The abundance of intratumoral bacteria in breast cancer indicates their potential role in its development and progression[4]. Understanding the diverse roles played by different bacteria could uncover novel insights into carcinogenic mechanisms and relationships. Investigating intratumoral bacteria represents an exciting new frontier in cancer research, with future findings on this topic poised to significantly contribute to our understanding of cancer mechanisms and treatment.
Accurate determination of the spatiotemporal dynamics of interacting proteins during host cell-pathogen communication is crucial for understanding their biological and pathological functions. Recent success in the use of spectral imaging further demonstrates the feasibility of directly visualizing multiple molecular interactions within cellular structures during the progression of pathogen‒host cell interactions[57].
The establishment of enteric infection in a 3D organoid model system provided a valuable platform for screening targeted therapeutics for pathogen entry into host tumor cells for susceptibility. The 3D model of viruses is an excellent reference for the model system we could build for intratumoral bacteria. To delineate how host cell polarity regulates pathogen infectivity and how host genetic factors contribute to SARS-CoV-2 infection, we established 3D human gastric organoids for real-time imaging of the cellular response to enteric infection[3]. In this 3D model system, enteric infection can be mimicked using intraluminal microinjection by which pathogens and their metabolites can enter the host cell via the apical membrane (Fig. 4)[58]. This type of study has revealed the primary and secondary entries of SARS-CoV-2 infection in gastric organoids and their spatiotemporal dynamics on cytokine secretion. There is no doubt that consolidation of the protein‒protein interaction network and circuitry underlying pathogen‒host communications combined with high-resolution illumination of molecular dynamics will enable us to interrogate the pathogenesis and progression of tumors.
Intratumoral bacteria have the potential to become new tools for diagnosing cancer. Poore et al.[59] re-examined whole-genome and whole-transcriptome sequencing studies in TCGA of 33 types of cancer from treatment-naive patients, detecting microbial reads and identifying unique microbial signatures in tumoral tissue and blood. By normalizing TCGA data, they suggested a new model for diagnostic tools that supplements existing ctDNA assays, allowing for detecting and monitoring cancer by predicting types for cancers, measuring contamination of cancer and checking bacterial signatures in blood.
Recent progress in synthetic biology tools represents an opportunity to repurpose bacteria as tumor-specific delivery systems given that microbes colonize and reside in tumors[60]. A better understanding of the dynamic molecular society of tumor cells orchestrated by intratumoral bacteria will enable us to engineer therapeutic microbes for precision and personalized medicine.
This work was supported by the National Key Research and Development Program of China (2022YFA1303100, 2022YFA0806800), the National Natural Science Foundation of China (92254302, 92153302), Plans for Major Provincial Science & Technology Projects of Anhui Province (202303a0702003), the Ministry of Education Innovative Team (IRT_17R102), and the Fundamental Research Funds for the Central Universities (WK2070000194).
The authors declare that they have no conflict of interest.
[1] |
de Martel C, Ferlay J, Franceschi S, et al. Global burden of cancers attributable to infections in 2008: a review and synthetic analysis. The Lancet Oncology, 2012, 13 (6): 607–615. DOI: 10.1016/S1470-2045(12)70137-7
|
[2] |
Sfanos K S, Sauvageot J, Fedor H L, et al. A molecular analysis of prokaryotic and viral DNA sequences in prostate tissue from patients with prostate cancer indicates the presence of multiple and diverse microorganisms. The Prostate, 2008, 68 (3): 306–320. DOI: 10.1002/pros.20680
|
[3] |
Yao X, Smolka A J. Gastric parietal cell physiology and Helicobacter pylori –induced disease. Gastroenterology, 2019, 156 (8): 2158–2173. DOI: 10.1053/j.gastro.2019.02.036
|
[4] |
Nejman D, Livyatan I, Fuks G, et al. The human tumor microbiome is composed of tumor type-specific intracellular bacteria. Science, 2020, 368 (6494): 973–980. DOI: 10.1126/science.aay9189
|
[5] |
Fu A K, Yao B Q, Dong T T, et al. Tumor-resident intracellular microbiota promotes metastatic colonization in breast cancer. Cell, 2022, 185 (8): 1356–1372. DOI: 10.1016/j.cell.2022.02.027
|
[6] |
Longmore G D. Bacteria in tumors “hit the road” together. Cell, 2022, 185 (8): 1292–1294. DOI: 10.1016/j.cell.2022.03.013
|
[7] |
Garrett W S. Cancer and the microbiota. Science, 2015, 348 (6230): 80–86. DOI: 10.1126/science.aaa4972
|
[8] |
Chiu C Y. Viral pathogen discovery. Current Opinion in Microbiology, 2013, 16 (4): 468–478. DOI: 10.1016/j.mib.2013.05.001
|
[9] |
Parsonnet J. Bacterial infection as a cause of cancer. Environmental Health Perspectives, 1995, 103 (Suppl 8): 263–268. DOI: 10.1289/ehp.95103s8263
|
[10] |
IARC Working Group on the Evaluation of Carcinogenic Risks to Humans. Schistosomes, Liver Flukes and Helicobacter pylori. IARC Monographs on the Evaluation of Carcinogenic Risks to Humans, 1994 , 61: 177–240.
|
[11] |
Payne R J H, Nowak M A, Blumberg B S. Analysis of a cellular model to account for the natural history of infection by the hepatitis B virus and its role in the development of primary hepatocellular carcinoma. Journal of Theoretical Biology, 1992, 159 (2): 215–240. DOI: 10.1016/S0022-5193(05)80703-9
|
[12] |
Rosin M P, El Din Zaki S S, Ward A J, et al. Involvement of inflammatory reactions and elevated cell proliferation in the development of bladder cancer in schistosomiasis patients. Mutation Research-Fundamental and Molecular Mechanisms of Mutagenesis, 1994, 305 (2): 283–292. DOI: 10.1016/0027-5107(94)90248-8
|
[13] |
Hirano S, Nakama R, Tamaki M, et al. Isolation and characterization of thirteen intestinal microorganisms capable of 7 alpha-dehydroxylating bile acids. Applied and Environmental Microbiology, 1981, 41 (3): 737–745. DOI: 10.1128/aem.41.3.737-745.1981
|
[14] |
Stadler J, Yeung K S, Furrer R, et al. Proliferative activity of rectal mucosa and soluble fecal bile acids in patients with normal colons and in patients with colonic polyps or cancer. Cancer Letters, 1988, 38 (3): 315–320. DOI: 10.1016/0304-3835(88)90023-7
|
[15] |
Hill M J. Bile acids and colorectal cancer: hypothesis. European Journal of Cancer Prevention, 1991, 1 (Suppl 2): 69–74. DOI: 10.1097/00008469-199110002-00012
|
[16] |
Baptista J, Bruce W R, Gupta I, et al. On distribution of different fecapentaenes, the fecal mutagens, in the human population. Cancer Letters, 1984, 22 (3): 299–303. DOI: 10.1016/0304-3835(84)90166-6
|
[17] |
Povey A C, Schiffman M, Taffe B G, et al. Laboratory and epidemiologic studies of fecapentaenes. Mutation Research, 1991, 259 (3/4): 387–397. DOI: 10.1016/0165-1218(91)90129-a
|
[18] |
Gupta I, Baptista J, Bruce W R, et al. Structures of fecapentaenes, the mutagens of bacterial origin isolated from human feces. Biochemistry, 1983, 22 (2): 241–245. DOI: 10.1021/bi00271a001
|
[19] |
Deschner E E, Ruperto J F, Lupton J R, et al. Dietary butyrate (tributyrin) does not enhance AOM-induced colon tumorigenesis. Cancer Letters, 1990, 52 (1): 79–82. DOI: 10.1016/0304-3835(90)90080-H
|
[20] |
Mcintyre A, Gibson P R, Young G P. Butyrate production from dietary fibre and protection against large bowel cancer in a rat model. Gut, 1993, 34 (3): 386–391. DOI: 10.1136/gut.34.3.386
|
[21] |
Yu T C, Guo F F, Yu Y N, et al. Fusobacterium nucleatum promotes chemoresistance to colorectal cancer by modulating autophagy. Cell, 2017, 170 (3): 548–563. DOI: 10.1016/j.cell.2017.07.008
|
[22] |
Geller L T, Barzily-Rokni M, Danino T, et al. Potential role of intratumor bacteria in mediating tumor resistance to the chemotherapeutic drug gemcitabine. Science, 2017, 357 (6356): 1156–1160. DOI: 10.1126/science.aah5043
|
[23] |
Hoption Cann S A, van Netten J P, van Netten C. Dr William Coley and tumour regression: a place in history or in the future. Postgraduate Medical Journal, 2003, 79 (938): 672–680. DOI: 10.1093/postgradmedj/79.938.672
|
[24] |
Mager D L. Bacteria and cancer: cause, coincidence or cure? A review. Journal of translational medicine, 2006, 4 (1): 14. DOI: 10.1186/1479-5876-4-14
|
[25] |
de Jong R, Altare F, Haagen I A, et al. Severe mycobacterial and Salmonella infections in interleukin-12 receptor-deficient patients. Science, 1998, 280 (5368): 1435–1438. DOI: 10.1126/science.280.5368.1435
|
[26] |
Flemer B, Lynch D B, Brown J M R, et al. Tumour-associated and non-tumour-associated microbiota in colorectal cancer. Gut, 2017, 66 (4): 633–643. DOI: 10.1136/gutjnl-2015-309595
|
[27] |
Haggar F A, Boushey R P. Colorectal cancer epidemiology: incidence, mortality, survival, and risk factors. Clinics in Colon and Rectal Surgery, 2009, 22 (4): 191–197. DOI: 10.1055/s-0029-1242458
|
[28] |
Zackular J P, Rogers M A M, Ruffin M T, et al. The human gut microbiome as a screening tool for colorectal cancer. Cancer Prevention Research, 2014, 7 (11): 1112–1121. DOI: 10.1158/1940-6207.CAPR-14-0129
|
[29] |
Yu J, Feng Q, Wong S H, et al. Metagenomic analysis of faecal microbiome as a tool towards targeted non-invasive biomarkers for colorectal cancer. Gut, 2017, 66 (1): 70–78. DOI: 10.1136/gutjnl-2015-309800
|
[30] |
Zeller G, Tap J, Voigt A Y, et al. Potential of fecal microbiota for early-stage detection of colorectal cancer. Molecular Systems Biology, 2014, 10 (11): 766. DOI: 10.15252/msb.20145645
|
[31] |
Galeano Niño J L, Wu H, LaCourse K D, et al. Effect of the intratumoral microbiota on spatial and cellular heterogeneity in cancer. Nature, 2022, 611 (7937): 810–817. DOI: 10.1038/s41586-022-05435-0
|
[32] |
Provenzano P P, Eliceiri K W, Campbell J M, et al. Collagen reorganization at the tumor-stromal interface facilitates local invasion. BMC Medicine, 2006, 4 (1): 38. DOI: 10.1186/1741-7015-4-38
|
[33] |
Conklin M W, Eickhoff J C, Riching K M, et al. Aligned collagen is a prognostic signature for survival in human breast carcinoma. The American Journal of Pathology, 2011, 178 (3): 1221–1232. DOI: 10.1016/j.ajpath.2010.11.076
|
[34] |
Ingman W V, Wyckoff J, Gouon-Evans V, et al. Macrophages promote collagen fibrillogenesis around terminal end buds of the developing mammary gland. Developmental Dynamics, 2006, 235 (12): 3222–3229. DOI: 10.1002/dvdy.20972
|
[35] |
Leek R D, Harris A L. Tumor-associated macrophages in breast cancer. Journal of Mammary Gland Biology and Neoplasia, 2002, 7 (2): 177–189. DOI: 10.1023/A:1020304003704
|
[36] |
Chen J Q, Yao Y D, Gong C, et al. CCL18 from tumor-associated macrophages promotes breast cancer metastasis via PITPNM3. Cancer Cell, 2011, 19 (4): 541–555. DOI: 10.1016/j.ccr.2011.02.006
|
[37] |
Xu H X, Lyu X D, Yi M, et al. Organoid technology and applications in cancer research. Journal of Hematology & Oncology, 2018, 11 (1): 116. DOI: 10.1186/s13045-018-0662-9
|
[38] |
Ness R B, Cauley J A. Antibiotics and breast cancer—what’s the meaning of this? JAMA, 2004, 291 (7): 880–881. DOI: 10.1001/jama.291.7.880
|
[39] |
Rao V P, Poutahidis T, Fox J G, et al. Breast cancer: should gastrointestinal bacteria be on our radar screen? Cancer Research, 2007, 67 (3): 847–850. DOI: 10.1158/0008-5472.CAN-06-3468
|
[40] |
Kalaora S, Nagler A, Nejman D, et al. Identification of bacteria-derived HLA-bound peptides in melanoma. Nature, 2021, 592 (7852): 138–143. DOI: 10.1038/s41586-021-03368-8
|
[41] |
Strobel M, Pförtner H, Tuchscherr L, et al. Post-invasion events after infection with Staphylococcus aureus are strongly dependent on both the host cell type and the infecting S. aureus strain. Clinical Microbiology and Infection, 2016, 22 (9): 799–809. DOI: 10.1016/j.cmi.2016.06.020
|
[42] |
Urbaniak C, Gloor G B, Brackstone M, et al. The microbiota of breast tissue and its association with breast cancer. Applied and Environmental Microbiology, 2016, 82 (16): 5039–5048. DOI: 10.1128/AEM.01235-16
|
[43] |
Buc E, Dubois D, Sauvanet P, et al. High prevalence of mucosa-associated E. coli producing cyclomodulin and genotoxin in colon cancer. PLoS ONE, 2013, 8 (2): e56964. DOI: 10.1371/journal.pone.0056964
|
[44] |
Cuevas-Ramos G, Petit C R, Marcq I, et al. Escherichia coli induces DNA damage in vivo and triggers genomic instability in mammalian cells. Proceedings of the National Academy of Sciences of the United States of America, 2010, 107 (25): 11537–11542. DOI: 10.1073/pnas.1001261107
|
[45] |
Arthur J C, Perez-Chanona E, Mühlbauer M, et al. Intestinal inflammation targets cancer-inducing activity of the microbiota. Science, 2012, 338 (6103): 120–123. DOI: 10.1126/science.1224820
|
[46] |
Nougayrède J P, Homburg S, Taieb F, et al. Escherichia coli induces DNA double-strand breaks in eukaryotic cells. Science, 2006, 313 (5788): 848–851. DOI: 10.1126/science.1127059
|
[47] |
Fernández M F, Reina-Pérez I, Astorga J M, et al. Breast cancer and its relationship with the microbiota. International Journal of Environmental Research and Public Health, 2018, 15 (8): 1747. DOI: 10.3390/ijerph15081747
|
[48] |
Hieken T J, Chen J, Hoskin T L, et al. The microbiome of aseptically collected human breast tissue in benign and malignant disease. Scientific Reports, 2016, 6 (1): 30751. DOI: 10.1038/srep30751
|
[49] |
Xue T, Lu Y, Yang H, et al. Isothermal RNA amplification for the detection of viable pathogenic bacteria to estimate the Salmonella virulence for causing enteritis. Journal of Agricultural and Food Chemistry, 2022, 70 (5): 1670–1678. DOI: 10.1021/acs.jafc.1c07182
|
[50] |
Urbaniak C, Cummins J, Brackstone M, et al. Microbiota of human breast tissue. Applied and Environmental Microbiology, 2014, 80 (10): 3007–3014. DOI: 10.1128/AEM.00242-14
|
[51] |
Thompson K J, Ingle J N, Tang X, et al. A comprehensive analysis of breast cancer microbiota and host gene expression. PLoS ONE, 2017, 12 (11): e0188873. DOI: 10.1371/journal.pone.0188873
|
[52] |
Ghoncheh M, Pournamdar Z, Salehiniya H. Incidence and mortality and epidemiology of breast cancer in the world. Asian Pacific Journal of Cancer Prevention, 2016, 17 (sup3): 43–46. DOI: 10.7314/APJCP.2016.17.S3.43
|
[53] |
Soto A M, Sonnenschein C. Environmental causes of cancer: endocrine disruptors as carcinogens. Nature Reviews Endocrinology, 2010, 6 (7): 363–370. DOI: 10.1038/nrendo.2010.87
|
[54] |
Goering P L, Aposhian H V, Mass M J, et al. The enigma of arsenic carcinogenesis: role of metabolism. Toxicological Sciences, 1999, 49 (1): 5–14. DOI: 10.1093/toxsci/49.1.5
|
[55] |
Sorahan T, Harrington J M. Lung cancer in Yorkshire chrome platers, 1972–97. Occupational & Environmental Medicine, 2000, 57 (6): 385–389. DOI: 10.1136/oem.57.6.385
|
[56] |
Lei Y X, Chen X M, Chen J K. Antisense translation elongation factor TEF_1δ reverses the carcinogenicity of cadmium chloride. Carcinogenesis, Teratogenesis and Mutagenesis, 2005, 17 (1): 1–4.
|
[57] |
Liu X, Liu X, Wang H, et al. Phase separation drives decision making in cell division. Journal of Biological Chemistry, 2020, 295 (39): 13419–13431. DOI: 10.1074/jbc.REV120.011746
|
[58] |
Wang W, Yang F, Lin J, et al. Modeling of COVID-19 disease disparity in gastric organoids reveals the spatiotemporal dynamics of SARS-CoV-2 infectivity. Journal of Molecular Cell Biology, 2022, 14 (2): mjac007. DOI: 10.1093/jmcb/mjac007
|
[59] |
Poore G D, Kopylova E, Zhu Q, et al. Microbiome analyses of blood and tissues suggest cancer diagnostic approach. Nature, 2020, 579 (7800): 567–574. DOI: 10.1038/s41586-020-2095-1
|
[60] |
Gurbatri C R, Arpaia N, Danino T. Engineering bacteria as interactive cancer therapies. Science, 2022, 378 (6622): 858–864. DOI: 10.1126/science.add9667
|
Figure 1. Three modes of how bacteria weaken cancer. (a) Bacterial colonization can lower the risk of certain cancers by either impeding the production of cancer cells or occupying the space where tumors typically grow. (b) If bacterial colonization is absent, ample space and nutrition could be available for cancer cells to thrive. (c) Either the bacteria themselves or their toxins have the ability to affect cancer cells, potentially eliminating or weakening them.
Figure 2. A hypothetical model of bacterium-induced breast cancer. Breast cancer in mice occurs from infection with H. hepaticus bacteria that stem from mammary ducts, inflammatory foci and proliferative epithelium of ducts. The spectrum of morphological intermediates from normal breast tissue (A) to precancerous (B and C) and tumor (D) states are shown: ductal hyperplasia (B) with focal alveolar hyperplasia (B, insets), early adenosquamous metaplasia (C) with ductal carcinoma in situ (breast intraepithelial neoplasia) and apocrine cytoplasmic differentiation (C, insets), and finally adenocarcinoma (D). H&E staining. Magnification, 40× (A–D); 400× (insets). Adapted with permission from Ref. [39]. Copyright 2007, American Association for Cancer Research.
Figure 3. Bacterial specificity in certain tumor types. The green circle signifies that these bacteria have a noticeable enrichment in certain tumor types. By examining the list, it is apparent that breast cancer cells harbor significantly more diverse bacterial species than other tumors, suggesting that they could be suitable candidates for studying intratumoral bacteria. It only highlights the specificity of bacterial species and does not indicate their prevalence in the original article.
Figure 4. A representative model of bacterial entry into host cells using 3D organoids. Molecular delineation of primary and secondary infection could offer a novel niche to learn about virus‒host interactions for disease disparity and severity. Adapted from Ref. [58]. Copyright 2022, The Author(s).
[1] |
de Martel C, Ferlay J, Franceschi S, et al. Global burden of cancers attributable to infections in 2008: a review and synthetic analysis. The Lancet Oncology, 2012, 13 (6): 607–615. DOI: 10.1016/S1470-2045(12)70137-7
|
[2] |
Sfanos K S, Sauvageot J, Fedor H L, et al. A molecular analysis of prokaryotic and viral DNA sequences in prostate tissue from patients with prostate cancer indicates the presence of multiple and diverse microorganisms. The Prostate, 2008, 68 (3): 306–320. DOI: 10.1002/pros.20680
|
[3] |
Yao X, Smolka A J. Gastric parietal cell physiology and Helicobacter pylori –induced disease. Gastroenterology, 2019, 156 (8): 2158–2173. DOI: 10.1053/j.gastro.2019.02.036
|
[4] |
Nejman D, Livyatan I, Fuks G, et al. The human tumor microbiome is composed of tumor type-specific intracellular bacteria. Science, 2020, 368 (6494): 973–980. DOI: 10.1126/science.aay9189
|
[5] |
Fu A K, Yao B Q, Dong T T, et al. Tumor-resident intracellular microbiota promotes metastatic colonization in breast cancer. Cell, 2022, 185 (8): 1356–1372. DOI: 10.1016/j.cell.2022.02.027
|
[6] |
Longmore G D. Bacteria in tumors “hit the road” together. Cell, 2022, 185 (8): 1292–1294. DOI: 10.1016/j.cell.2022.03.013
|
[7] |
Garrett W S. Cancer and the microbiota. Science, 2015, 348 (6230): 80–86. DOI: 10.1126/science.aaa4972
|
[8] |
Chiu C Y. Viral pathogen discovery. Current Opinion in Microbiology, 2013, 16 (4): 468–478. DOI: 10.1016/j.mib.2013.05.001
|
[9] |
Parsonnet J. Bacterial infection as a cause of cancer. Environmental Health Perspectives, 1995, 103 (Suppl 8): 263–268. DOI: 10.1289/ehp.95103s8263
|
[10] |
IARC Working Group on the Evaluation of Carcinogenic Risks to Humans. Schistosomes, Liver Flukes and Helicobacter pylori. IARC Monographs on the Evaluation of Carcinogenic Risks to Humans, 1994 , 61: 177–240.
|
[11] |
Payne R J H, Nowak M A, Blumberg B S. Analysis of a cellular model to account for the natural history of infection by the hepatitis B virus and its role in the development of primary hepatocellular carcinoma. Journal of Theoretical Biology, 1992, 159 (2): 215–240. DOI: 10.1016/S0022-5193(05)80703-9
|
[12] |
Rosin M P, El Din Zaki S S, Ward A J, et al. Involvement of inflammatory reactions and elevated cell proliferation in the development of bladder cancer in schistosomiasis patients. Mutation Research-Fundamental and Molecular Mechanisms of Mutagenesis, 1994, 305 (2): 283–292. DOI: 10.1016/0027-5107(94)90248-8
|
[13] |
Hirano S, Nakama R, Tamaki M, et al. Isolation and characterization of thirteen intestinal microorganisms capable of 7 alpha-dehydroxylating bile acids. Applied and Environmental Microbiology, 1981, 41 (3): 737–745. DOI: 10.1128/aem.41.3.737-745.1981
|
[14] |
Stadler J, Yeung K S, Furrer R, et al. Proliferative activity of rectal mucosa and soluble fecal bile acids in patients with normal colons and in patients with colonic polyps or cancer. Cancer Letters, 1988, 38 (3): 315–320. DOI: 10.1016/0304-3835(88)90023-7
|
[15] |
Hill M J. Bile acids and colorectal cancer: hypothesis. European Journal of Cancer Prevention, 1991, 1 (Suppl 2): 69–74. DOI: 10.1097/00008469-199110002-00012
|
[16] |
Baptista J, Bruce W R, Gupta I, et al. On distribution of different fecapentaenes, the fecal mutagens, in the human population. Cancer Letters, 1984, 22 (3): 299–303. DOI: 10.1016/0304-3835(84)90166-6
|
[17] |
Povey A C, Schiffman M, Taffe B G, et al. Laboratory and epidemiologic studies of fecapentaenes. Mutation Research, 1991, 259 (3/4): 387–397. DOI: 10.1016/0165-1218(91)90129-a
|
[18] |
Gupta I, Baptista J, Bruce W R, et al. Structures of fecapentaenes, the mutagens of bacterial origin isolated from human feces. Biochemistry, 1983, 22 (2): 241–245. DOI: 10.1021/bi00271a001
|
[19] |
Deschner E E, Ruperto J F, Lupton J R, et al. Dietary butyrate (tributyrin) does not enhance AOM-induced colon tumorigenesis. Cancer Letters, 1990, 52 (1): 79–82. DOI: 10.1016/0304-3835(90)90080-H
|
[20] |
Mcintyre A, Gibson P R, Young G P. Butyrate production from dietary fibre and protection against large bowel cancer in a rat model. Gut, 1993, 34 (3): 386–391. DOI: 10.1136/gut.34.3.386
|
[21] |
Yu T C, Guo F F, Yu Y N, et al. Fusobacterium nucleatum promotes chemoresistance to colorectal cancer by modulating autophagy. Cell, 2017, 170 (3): 548–563. DOI: 10.1016/j.cell.2017.07.008
|
[22] |
Geller L T, Barzily-Rokni M, Danino T, et al. Potential role of intratumor bacteria in mediating tumor resistance to the chemotherapeutic drug gemcitabine. Science, 2017, 357 (6356): 1156–1160. DOI: 10.1126/science.aah5043
|
[23] |
Hoption Cann S A, van Netten J P, van Netten C. Dr William Coley and tumour regression: a place in history or in the future. Postgraduate Medical Journal, 2003, 79 (938): 672–680. DOI: 10.1093/postgradmedj/79.938.672
|
[24] |
Mager D L. Bacteria and cancer: cause, coincidence or cure? A review. Journal of translational medicine, 2006, 4 (1): 14. DOI: 10.1186/1479-5876-4-14
|
[25] |
de Jong R, Altare F, Haagen I A, et al. Severe mycobacterial and Salmonella infections in interleukin-12 receptor-deficient patients. Science, 1998, 280 (5368): 1435–1438. DOI: 10.1126/science.280.5368.1435
|
[26] |
Flemer B, Lynch D B, Brown J M R, et al. Tumour-associated and non-tumour-associated microbiota in colorectal cancer. Gut, 2017, 66 (4): 633–643. DOI: 10.1136/gutjnl-2015-309595
|
[27] |
Haggar F A, Boushey R P. Colorectal cancer epidemiology: incidence, mortality, survival, and risk factors. Clinics in Colon and Rectal Surgery, 2009, 22 (4): 191–197. DOI: 10.1055/s-0029-1242458
|
[28] |
Zackular J P, Rogers M A M, Ruffin M T, et al. The human gut microbiome as a screening tool for colorectal cancer. Cancer Prevention Research, 2014, 7 (11): 1112–1121. DOI: 10.1158/1940-6207.CAPR-14-0129
|
[29] |
Yu J, Feng Q, Wong S H, et al. Metagenomic analysis of faecal microbiome as a tool towards targeted non-invasive biomarkers for colorectal cancer. Gut, 2017, 66 (1): 70–78. DOI: 10.1136/gutjnl-2015-309800
|
[30] |
Zeller G, Tap J, Voigt A Y, et al. Potential of fecal microbiota for early-stage detection of colorectal cancer. Molecular Systems Biology, 2014, 10 (11): 766. DOI: 10.15252/msb.20145645
|
[31] |
Galeano Niño J L, Wu H, LaCourse K D, et al. Effect of the intratumoral microbiota on spatial and cellular heterogeneity in cancer. Nature, 2022, 611 (7937): 810–817. DOI: 10.1038/s41586-022-05435-0
|
[32] |
Provenzano P P, Eliceiri K W, Campbell J M, et al. Collagen reorganization at the tumor-stromal interface facilitates local invasion. BMC Medicine, 2006, 4 (1): 38. DOI: 10.1186/1741-7015-4-38
|
[33] |
Conklin M W, Eickhoff J C, Riching K M, et al. Aligned collagen is a prognostic signature for survival in human breast carcinoma. The American Journal of Pathology, 2011, 178 (3): 1221–1232. DOI: 10.1016/j.ajpath.2010.11.076
|
[34] |
Ingman W V, Wyckoff J, Gouon-Evans V, et al. Macrophages promote collagen fibrillogenesis around terminal end buds of the developing mammary gland. Developmental Dynamics, 2006, 235 (12): 3222–3229. DOI: 10.1002/dvdy.20972
|
[35] |
Leek R D, Harris A L. Tumor-associated macrophages in breast cancer. Journal of Mammary Gland Biology and Neoplasia, 2002, 7 (2): 177–189. DOI: 10.1023/A:1020304003704
|
[36] |
Chen J Q, Yao Y D, Gong C, et al. CCL18 from tumor-associated macrophages promotes breast cancer metastasis via PITPNM3. Cancer Cell, 2011, 19 (4): 541–555. DOI: 10.1016/j.ccr.2011.02.006
|
[37] |
Xu H X, Lyu X D, Yi M, et al. Organoid technology and applications in cancer research. Journal of Hematology & Oncology, 2018, 11 (1): 116. DOI: 10.1186/s13045-018-0662-9
|
[38] |
Ness R B, Cauley J A. Antibiotics and breast cancer—what’s the meaning of this? JAMA, 2004, 291 (7): 880–881. DOI: 10.1001/jama.291.7.880
|
[39] |
Rao V P, Poutahidis T, Fox J G, et al. Breast cancer: should gastrointestinal bacteria be on our radar screen? Cancer Research, 2007, 67 (3): 847–850. DOI: 10.1158/0008-5472.CAN-06-3468
|
[40] |
Kalaora S, Nagler A, Nejman D, et al. Identification of bacteria-derived HLA-bound peptides in melanoma. Nature, 2021, 592 (7852): 138–143. DOI: 10.1038/s41586-021-03368-8
|
[41] |
Strobel M, Pförtner H, Tuchscherr L, et al. Post-invasion events after infection with Staphylococcus aureus are strongly dependent on both the host cell type and the infecting S. aureus strain. Clinical Microbiology and Infection, 2016, 22 (9): 799–809. DOI: 10.1016/j.cmi.2016.06.020
|
[42] |
Urbaniak C, Gloor G B, Brackstone M, et al. The microbiota of breast tissue and its association with breast cancer. Applied and Environmental Microbiology, 2016, 82 (16): 5039–5048. DOI: 10.1128/AEM.01235-16
|
[43] |
Buc E, Dubois D, Sauvanet P, et al. High prevalence of mucosa-associated E. coli producing cyclomodulin and genotoxin in colon cancer. PLoS ONE, 2013, 8 (2): e56964. DOI: 10.1371/journal.pone.0056964
|
[44] |
Cuevas-Ramos G, Petit C R, Marcq I, et al. Escherichia coli induces DNA damage in vivo and triggers genomic instability in mammalian cells. Proceedings of the National Academy of Sciences of the United States of America, 2010, 107 (25): 11537–11542. DOI: 10.1073/pnas.1001261107
|
[45] |
Arthur J C, Perez-Chanona E, Mühlbauer M, et al. Intestinal inflammation targets cancer-inducing activity of the microbiota. Science, 2012, 338 (6103): 120–123. DOI: 10.1126/science.1224820
|
[46] |
Nougayrède J P, Homburg S, Taieb F, et al. Escherichia coli induces DNA double-strand breaks in eukaryotic cells. Science, 2006, 313 (5788): 848–851. DOI: 10.1126/science.1127059
|
[47] |
Fernández M F, Reina-Pérez I, Astorga J M, et al. Breast cancer and its relationship with the microbiota. International Journal of Environmental Research and Public Health, 2018, 15 (8): 1747. DOI: 10.3390/ijerph15081747
|
[48] |
Hieken T J, Chen J, Hoskin T L, et al. The microbiome of aseptically collected human breast tissue in benign and malignant disease. Scientific Reports, 2016, 6 (1): 30751. DOI: 10.1038/srep30751
|
[49] |
Xue T, Lu Y, Yang H, et al. Isothermal RNA amplification for the detection of viable pathogenic bacteria to estimate the Salmonella virulence for causing enteritis. Journal of Agricultural and Food Chemistry, 2022, 70 (5): 1670–1678. DOI: 10.1021/acs.jafc.1c07182
|
[50] |
Urbaniak C, Cummins J, Brackstone M, et al. Microbiota of human breast tissue. Applied and Environmental Microbiology, 2014, 80 (10): 3007–3014. DOI: 10.1128/AEM.00242-14
|
[51] |
Thompson K J, Ingle J N, Tang X, et al. A comprehensive analysis of breast cancer microbiota and host gene expression. PLoS ONE, 2017, 12 (11): e0188873. DOI: 10.1371/journal.pone.0188873
|
[52] |
Ghoncheh M, Pournamdar Z, Salehiniya H. Incidence and mortality and epidemiology of breast cancer in the world. Asian Pacific Journal of Cancer Prevention, 2016, 17 (sup3): 43–46. DOI: 10.7314/APJCP.2016.17.S3.43
|
[53] |
Soto A M, Sonnenschein C. Environmental causes of cancer: endocrine disruptors as carcinogens. Nature Reviews Endocrinology, 2010, 6 (7): 363–370. DOI: 10.1038/nrendo.2010.87
|
[54] |
Goering P L, Aposhian H V, Mass M J, et al. The enigma of arsenic carcinogenesis: role of metabolism. Toxicological Sciences, 1999, 49 (1): 5–14. DOI: 10.1093/toxsci/49.1.5
|
[55] |
Sorahan T, Harrington J M. Lung cancer in Yorkshire chrome platers, 1972–97. Occupational & Environmental Medicine, 2000, 57 (6): 385–389. DOI: 10.1136/oem.57.6.385
|
[56] |
Lei Y X, Chen X M, Chen J K. Antisense translation elongation factor TEF_1δ reverses the carcinogenicity of cadmium chloride. Carcinogenesis, Teratogenesis and Mutagenesis, 2005, 17 (1): 1–4.
|
[57] |
Liu X, Liu X, Wang H, et al. Phase separation drives decision making in cell division. Journal of Biological Chemistry, 2020, 295 (39): 13419–13431. DOI: 10.1074/jbc.REV120.011746
|
[58] |
Wang W, Yang F, Lin J, et al. Modeling of COVID-19 disease disparity in gastric organoids reveals the spatiotemporal dynamics of SARS-CoV-2 infectivity. Journal of Molecular Cell Biology, 2022, 14 (2): mjac007. DOI: 10.1093/jmcb/mjac007
|
[59] |
Poore G D, Kopylova E, Zhu Q, et al. Microbiome analyses of blood and tissues suggest cancer diagnostic approach. Nature, 2020, 579 (7800): 567–574. DOI: 10.1038/s41586-020-2095-1
|
[60] |
Gurbatri C R, Arpaia N, Danino T. Engineering bacteria as interactive cancer therapies. Science, 2022, 378 (6622): 858–864. DOI: 10.1126/science.add9667
|